The increasing consumption of resources is an ongoing problem. By 2050, the demand will reach a level where the equivalent of three Earths will be needed to meet human demand for natural resources [1]. The Architecture, Engineering, and Construction (AEC) industry plays a crucial role, as it is responsible for 40−60% of the global raw material extraction [2], [3] and 33% of the European Union’s (EU) total waste generation [4].
The lack of available material, End-of-Life (EoL), and recycling data is one of the main barriers to implementing Circular Economy (CE) methods [5], [6]. Recent research focuses on CE in construction by incorporating digital technologies into this process [7]. A transition to a circular system, including an emission assessment, especially in AEC, is necessary as it is responsible for about 38% of all CO2 emissions and has a major impact on resource depletion [8], [9]. The European Green Deal developed the “EU Taxonomy” classification system based on six environmental objectives, according to which the sustainability of investments is evaluated.
This paper presents the ongoing research to enable integrated assessment of circularity and environmental sustainability in the early design stage, focusing on residential buildings in Austria. Compared to existing solutions (isolated solutions for partial evaluations without communication options), a continuous data pipeline and system integration of different software modules is being pursued. By creating the so-called Circular Twin (CT) digital ecosystem based on the parts of Algorithm Aided Design (AAD), Building Information Modelling (BIM) and Virtual Reality (VR), this paper proposes a framework that aims to support decision-making to enable Circular Construction and integrate end-of-life concepts into the early design process.
The proposed framework supports traditional architectural planning, and by linking the geometry of the preliminary architectural design to the digital CT ecosystem, the broad application of the practice is strived for. In addition to AAD methods providing automated variant generation coupled to a BIM object database, algorithms are implemented to assess the building design’s circularity potential and environmental impact. The stakeholders’ decision-making process will be supported by visualising the assessments of different design variants in a VR platform, including user interaction using Virtual Agent (VA) technology. Thus, the digital ecosystem enables the implementation of CE principles and reaching of CE goals.
The concept of the CE has gained prominence as a promising strategy to address the challenges of resource depletion, environmental degradation, and waste generation in the AEC industry. Many definitions of sustainability and circular economy are available, and most differ just in nuances. A good overview of different definitions and the heritage of the two terms is presented in [10]. In current literature, sustainability is described as “a development that meets the needs of the present without compromising the ability of future generations to meet their own needs” [11]. And CE is “a regenerative system in which resource input and waste, emission, and energy leakage are minimised by slowing, closing, and narrowing material and energy loops” [10]. That means a holistic approach, including material efficiency, should always be accompanied by a life cycle assessment. A study from 2012 analyses synergies between the three pillars of sustainability (environmental, social, and economic) using a principal component analysis identifying the principal components capturing synergies (1 Product and Process Development, 2 Education and Social Economics and 3 Protection of Nature and Humans) between the three pillars, using an empirical approach of a questionnaire from ETH graduates. The study also discovered that integrating one pillar often conflicts with implementing another dimension [12]. The United Nations (UN) tries to target these problems, among others, with the UN Sustainable Development Goals (SDG) [13]. Investigating the economic effects of implementing CE with direct and indirect effects would be at least beneficial from a macroeconomic point of view [14]. Other identified benefits from investigating barriers and potentials of CE implementation in AEC are reducing business risk due to the disruption potential in raw material supply chains and attributable cost instabilities [15].
As a crucial part of CE, Waste Management developed a strategy to reduce waste production and the consumption of natural resources like materials and landfill space. The strategy defined the waste hierarchy already implemented in national law [16]. These principles were further developed into the circular economy principles, namely designing out of waste, educating against pollution, keeping products and materials in use, and regenerating natural systems, generally summarised as the 10R hierarchy [17].
These principles can be applied to the built environment through various strategies:
Adaptive Reuse and Retrofitting: The adaptive reuse and retrofitting of existing structures to extend their lifespan reduces the demand for new construction materials and minimises waste generation. This approach requires innovative design solutions that cater to changing user needs while preserving the original structure’s integrity.
Design for Disassembly: Incorporating design features that facilitate the disassembly and separation of components enables easier recycling and reutilization at the end of a building’s life cycle.
Materials Recycling: Implementing efficient materials separation and recycling processes can significantly reduce the extraction of virgin resources and divert construction and demolition waste from landfills [18].
Analysing the literature of the past decades led to the conclusion that barriers are present at different levels, whereby 37 significantly thematised barriers were identified, which can be divided into six categories according to PESTLE − Political, Economic, Social, Technological, Legal, and Environmental [19]. The most relevant barriers identified for the research presented in this paper are the designer’s leadership role, lack of stakeholders’ awareness of the benefits of circularity in construction, information gap, and accurate quantification of the environmental benefits. Based on a literature review and analysis, another study proposes a tier-list approach for waste upcycling as a strategic way for businesses to close the loop within their industrial processes and production chains. The authors highlight benefits for society and the environment, like tackling resource scarcity and waste production problems and conclude that macro-, meso- and micro-level barriers must be tackled to enable CE [20].
Therefore, digital tools to enable enhanced project planning, material tracking, and life-cycle and other assessments are mandatory for aiding the identification of CE opportunities and CE implementation in the AEC industry,
Building Information Modelling (BIM) is a suitable digital tool acting as a joint knowledge base for planning process stakeholders. BIM can be seen as a method to generate a digital model of buildings, including relevant data throughout the life cycle [21]. A distinction between geometric and non-geometric (alphanumeric) parameters is necessary. Geometric parameters control the dimensions of the elements, and alphanumeric parameters assign costs, LCA data, building physics or other information. In contrast, a digital twin is the digital image of an actual building for which an actual physical building is required. Through sensor technology and the linking of real and physical objects, it is possible to monitor and control processes in the building [22].
Incorporating algorithms to support digital twins in the built environment shows that their performance is helpful in complex design processes and in identifying precise sources of error [23]. Other studies point to the lack of effective links between parametric, generative, and digital tools and processes and their sharing [24], [25]. In particular, the standardisation of BIM-based parametric modelling is cited as patchy. Additionally, implementation-oriented research is deficient in fully integrating digital twins that support more efficient implementation throughout the building life cycle [26]. Nevertheless, implementing digital twins provides tremendous benefits in the CE context [27]. A great relevance lies in developing an effective combination of parametric in BIM processes to determine building reuse assessment [28].
Stakeholder collaboration and project visualisation may be crucial. VR is a powerful tool for the AEC industry to visualise and inspect structures that are yet to be built [29]. With the increase in the choice of end-user devices, Virtual Reality is seen as an innovative technology for collaboration and decision support in various application areas. However, combining the benefits of VR and BIM is challenging, especially in parametric design in BIM. The need to convert 3D data and meta information from BIM to VR software has been cited as a significant difficulty [30], [31]. However, solutions that allow BIM models to be visualised in VR without a conversion step have been published [30–32]. Another difficulty is to allow VR users to modify the parametrically generated BIM model directly in VR and see the result of the modification in real time. In previously published projects that combine generative design and VR, user interactions are often enabled by menus that allow users to configure parameters [32].
Experiences from past research show that users often struggle with the new interaction paradigm to grasp the presented information [33]. This paper presents research to solve this problem by adopting a novel approach that employs a user interface using an embodied intelligent Virtual Agent. It is a graphical representation that often looks like a human figure and is controlled by a computer algorithm [34]. VAs have been used in numerous application areas of VR research, such as psychology [35], [36] and healthcare [37], [38], but have not been extensively demonstrated in AEC applications of VR. To date, using a VA to link information based on BIM data has only been documented in one study [39].
The EU taxonomy impacts the construction and real estate industry as one of the largest resource-consuming sectors. Some building certification systems, such as DGNB and LEED, offer plug-ins for BIM to speed up the building certification process [40]. However, the implementation of EU taxonomy compliance and corresponding metrics are not yet embedded in any software and should be highlighted as an innovation-driving component for the presented research.
The probably most investigated assessment method regarding the environment sustainability evaluation of buildings is the Life Cycle Assessment (LCA), defined by the International Organization for Standardization (ISO), Standard 14040:2006 [41] and Standard 14044:2006 [42]. LCA involves a sequential procedure encompassing distinct phases: delineating system boundaries, developing the life cycle inventory, conducting impact assessments, and interpreting resultant outcomes. LCA can be used to analyse and improve the production process of specific materials or regional aspects. For example, steel production in Brazil was analysed, different improvement options were defined, and the potential improvements of the different measures were assessed [43]. In contrast to such an in-depth process analysis, the LCA of buildings differs since they depend on data from such process analyses, and the material composition of the end product (building) plays the main role.
In the context of the AEC industry, the regulatory Framework EN 15978:2011 [44] prescribes LCA protocols. However, practical utilisation remains limited due to the demanding nature of data acquisition and the substantial implementation efforts, as highlighted by IBO [45]. Despite this, the potential for BIM-driven evaluations exists, supported by various software tools. Nevertheless, these tools grapple with the challenges of acquiring and managing copious data, particularly in the initial planning phases [46].
Tackling the problem of benchmarking building typologies and analysing different variations of a building’s design, a method including the BIM Model, Integrated life-cycle assessment (LCA) tool and Machine Learning Algorithms were explored and developed [47]. Important to note is that LCAs differ regarding their spatial and temporal system boundaries. A method to reduce the environmental impact through summing up eco-indicators: Global Warming Potential (GWP), Acidification Potential (AP), and Primary Energy Input-non-renewable (PEI) within one building evaluation is offered by the Austrian Institute for Building and Ecology (IBO) [45], including the modules A1−A3 according to Environmental Product Declarations (EPD) and different applicable spatial system boundaries [45]. Assessing the environmental impact of material use is crucial from a holistic point of view. Still, the attention in research is drifting more and more toward assessing the circularity of buildings and components.
A circularity assessment framework for buildings is crucial to evaluate and enhance their sustainability by measuring and optimising resource efficiency material flows. Circular Economy Metrics and Indicators are essential for assessing, quantifying, and guiding the transition towards a circular economy within various sectors, including the built environment.
The Material Circularity Index by the Ellen MacArthur Foundation is a metric used to assess the circularity of materials within a system or a product’s life cycle. It quantifies the degree to which materials within a system contribute to circular economy principles by focusing on their ability to be reused, recycled, or repurposed instead of discarded as waste [48]. Another approach is the Urban Mining Index, which is used to quantify the potential and efficiency of extracting, recovering, and recycling materials from urban stock. This index assesses availability, reclamation potential, and use as secondary material [49].
Another assessment method is the EI10 index, which assigns every material a reuse/ recycling rate and categorisation of disposal and recycling. Based on this information, the elements are assessed and aggregated for the entire building assessment [50].
Overview of potential Circular Twin assessment methods
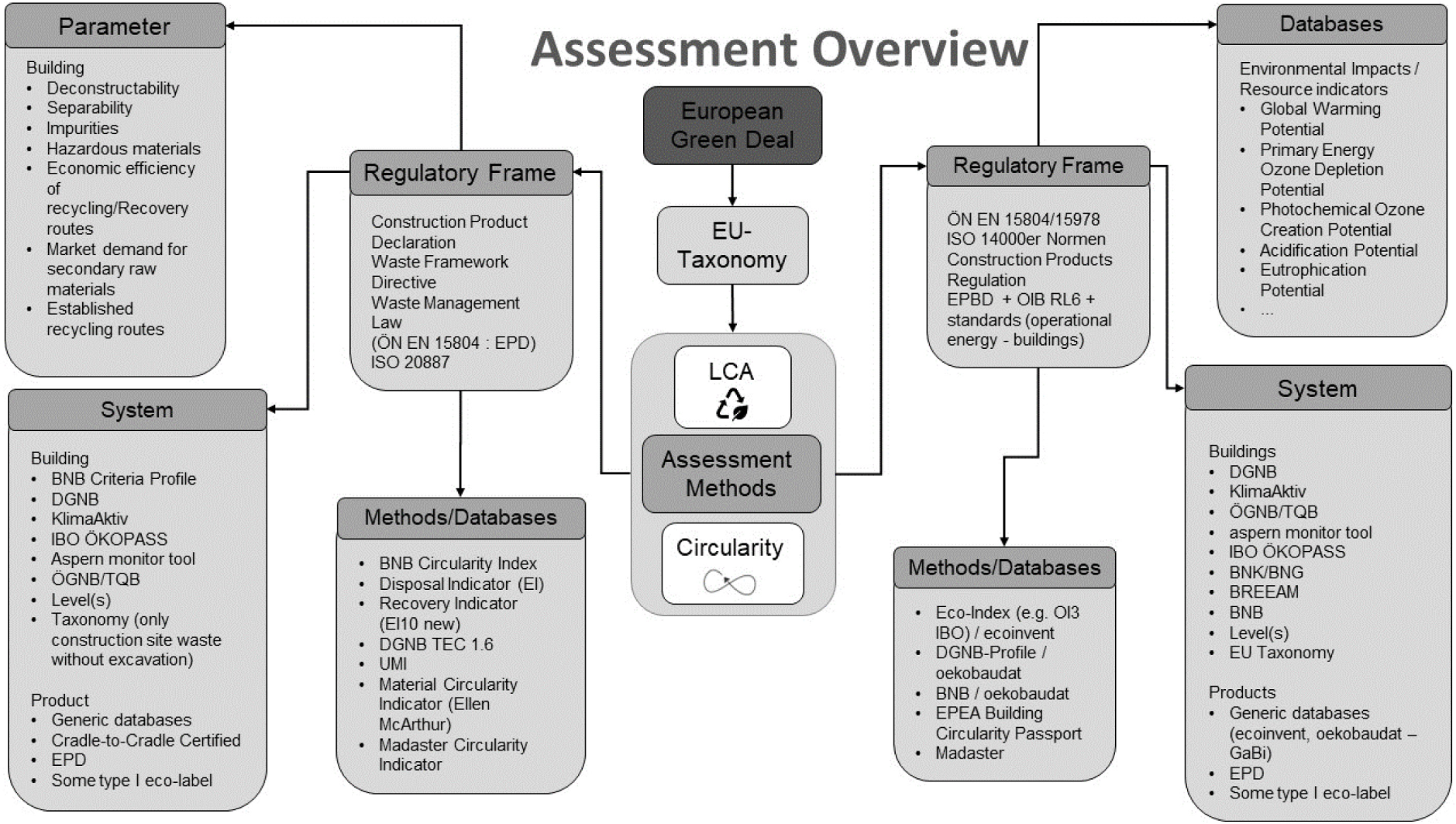
Tools that combine different approaches and methods are frequently called Material Passports (MPs), and different concepts have been developed in recent years [51]. MPs are a significant instrument for enhancing CE in the AEC industry. They serve as a document of the material characterisation/composition of a building and simultaneously offer the potential for building up digital material cadastres [38]. A BIM-based implementation is already possible, as shown in [52]. The approach is based on a study that presents the systematics of an MP, the information to include, and the importance of material knowledge to implement urban mining in practice [53]. Previous studies have shown that MPs are a suitable tool to evaluate material masses and can be used to improve deconstruction activities from a CE and economic point of view [54]. Weaknesses were identified in assessing the masses generated over the entire life cycle (resource requirements) and material flows (reuse and recycling rates). Based on different assessment methods and national legislation, a framework to assess the environmental impact and economic consequences of material circularity was developed for Austria [55]. The information required for that is also partly needed for creating MPs, emphasising the role of BIM and digital data storage.
BIM was identified as a suitable tool that can serve as a data storage tool and a data provider over a life cycle. Especially concerning CE, this is crucial, as reuse and recycling are severely limited or even impossible due to a lack of information. On the one hand, the environmental impact of construction measures is important for the environmental assessment, and on the other hand, for circularity assessment, as shown in Figure 1.
Resource use, emissions, and waste production affect the AEC industry’s role in the CE context. At the same time, waste management as part of a Circular Economy approach is seldom considered in the early design phase of buildings − the phase with the most significant impact on a building’s performance throughout the life cycle, thus the most significant influence through good design [56]. An easy-to-use assessment tool is needed to support stakeholders like planners and owners in decision-making. In order to reduce raw material extraction, it is of utmost importance to promote the reuse of building elements and high-quality recycling of materials, as well as prioritised principles such as extending service life to enable a CE. As there is a tension between architectural design and environmental and ecological sustainability, particularly in social housing, the tools are needed to enable collaborative and integrative planning methods.
Barriers to promoting CE are a lack of user awareness and knowledge deficit (limited skills, imagination, inspiration, and information). As an enabler to achieve CE, better mobilisation of knowledge was identified [20]. Therefore, benchmarking buildings will be crucial to promote sustainable building designs. A study investigating benchmarking in Spain identified the main problems: uncertainties regarding data quality and reliability of LCA data in general and the modification of LCA data, especially as the plug-in used in the study does not allow any adaptations. Furthermore, no periods of use could be read out [47].
The presented study tackled the problems mentioned above, as described in the next section. A connection is implemented between the Variant Study Module and the Virtual Reality Module to enable collaboration among all stakeholders. Furthermore, the study postulates using an extensive database, including material, operating data, and service times for materials and components; importing them to the project data module allows additional entries manually.
The research goal of the ongoing research project presented in this paper is to enable and evaluate the reusability, recyclability, and environmental impact of materials and components of a building over the whole life cycle already in the early planning phases. The aim is to couple architectural design, building sustainability assessment and stakeholder-collaboration platforms. This paper presents a framework proposal for generating and evaluating Circular Twins and visualising environmental sustainability assessment metrics in a VR environment, enabling the visualisation of a variant study of different building designs and collaboration between all stakeholders in the early design phase.
The Circular Twin framework is based on the architectural preliminary designs (BIM-Geometry); object databases enriched with circular economy indicators – the BIM4CE Object Database; generative algorithms that enable the automated variant study of assessed models (Circular Twins); as well as defined assessment-method; and result visualisation via VR platform that supports decision making via integrated VA. In this way, stakeholders are supported in the early design phases to review the concepts of reusability and recyclability or to integrate them as an inherent part of the design, thus significantly reducing construction waste, emissions and raw material consumption.
The proposed CT framework approaches the integration of commonly used BIM-planning processes with automated variant study and VR visualisation with minimal user interaction. Consistent data structuring, as the basis for model and data integration, will enable the coupling of the implemented framework displayed in Figure 2. It consists of eight parts, numbered as shown in the figure, merged into four modules described below.
Conceptual Circular Twin Framework – proposed Data Pipeline and Module Interfaces
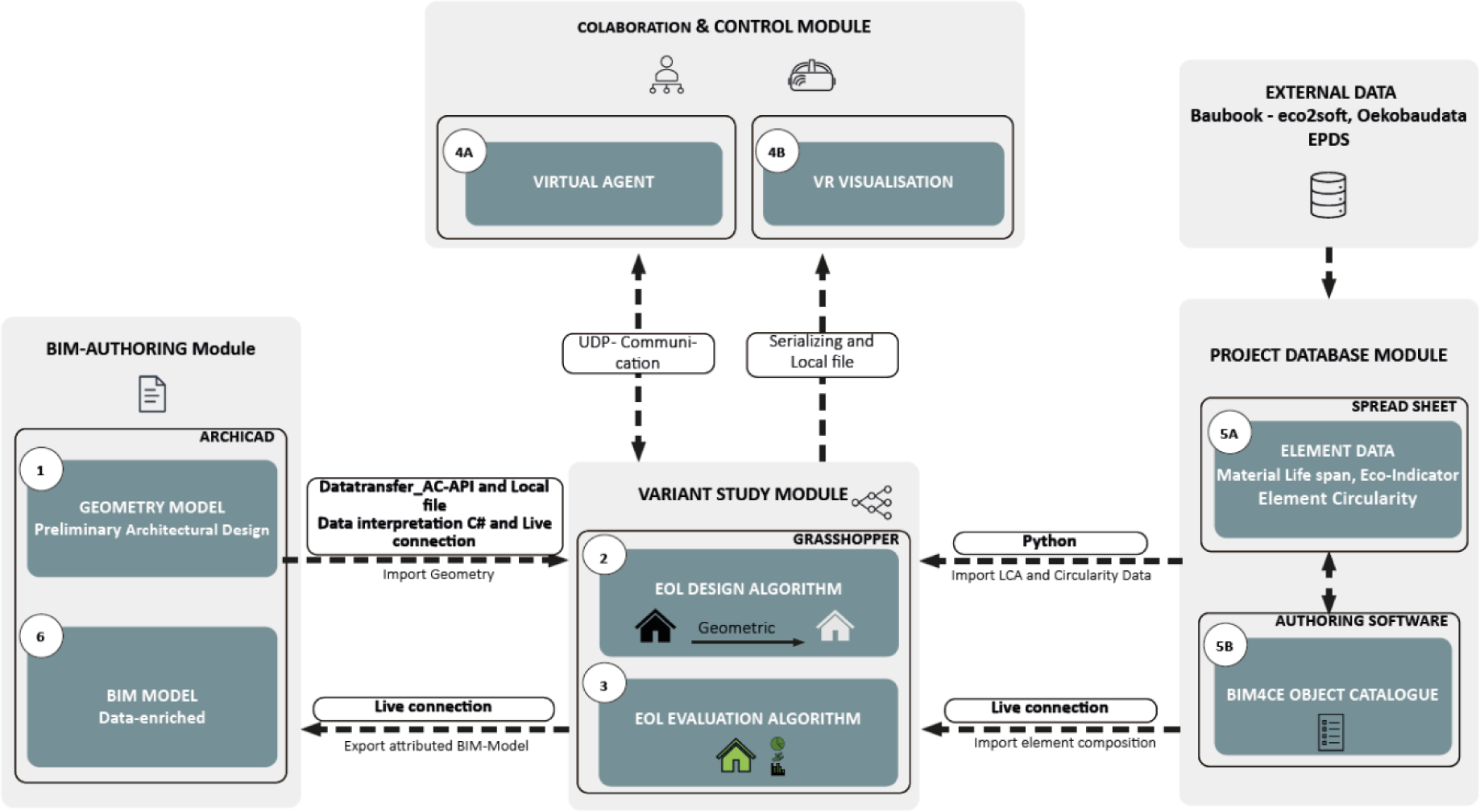
The BIM-Architectural Module (BA-M) consists of the Geometry Model and the BIM Model. Therefore, the data flow starts and ends simultaneously within the framework.
1) Geometry Model: The architectural design model in the form of an ArchiCAD file provides the geometry for the geometric twin. It makes no difference whether the model consists of single-layer or multi-layer elements. Essential, however, is that the elements have the correct element attribution, such as whether it is a wall or ceiling, or if they are load bearing or not, and that doors and windows are placed correctly. Further tests will include sketching tools and IFC as a geometry basis [57].
6) Building Information Modelling Model: The selected execution variant is translated into ArchiCAD elements and transferred to ArchiCAD via the live connection from Grasshopper. This model then forms the basis for further planning activities (detailed planning) and creating the digital twin once the structure has been built.
The Variant Study Module (VS-M) comprises the Design Algorithm and Evaluation Algorithm. It creates the Geometric Twin based on the preliminary Architectural Design from B-AM and assigns different attributed compositions to the elements, enabling the Assessment of different building designs. The chosen variant, including relevant data, can be passed on to BA-M.
2) Design Algorithm: The Geometric Twin generated in Rhino/Grasshopper based on basic geometric information via a (semi)bidirectional Live Connection or Archicad-API and direct model integration. The reconstruction of the architectural design is necessary because Grasshopper can not conduct basic operations with ArchiCAD elements. Therefore, geometric information needs to be filtered, and the model needs to be reconstructed. The variant study with the pre-defined composition is conducted using a Python script running a loop.
3) Evaluation Algorithm: includes potential parameters, building certifications, and assessment methods. The presented research includes LCA according to EU taxonomy, LCA according to Oi3-Index, and Circularity Index according to IBO. Geometric and material information are combined and made available to the calculation methods. The mass calculation and the assignment of the correct characteristic values are carried out automatically, enabling a variant study based on the selected observation space and ensuring rapid analysis when switching between variants and displaying the results in the VR environment.
The Collaboration and Control Module (CC-M) consists of two components: Virtual Reality Visualisation, showcasing the different variants and assessment metrics to enable collaborative processes, and the Virtual Agent, providing project-related information and controlling the VS-M.
4A) Virtual Reality Visualisation: The geometry generated in Grasshopper is efficiently transmitted to Unity through a serialisation and deserialisation process, ensuring rapid data transfer. Moreover, the bidirectional communication between Grasshopper and Unity is accomplished using User Datagram Protocol (UDP), enabling a seamless exchange of messages between the two software components. The VR platform serves as a visualisation tool for the building model and for displaying the assessment results.
4B) Virtual Agent: The integration of the VA allows queries and change requests to be made. These are, for example, queries on the CO2 footprint or circularity rating. Future queries will include analysing the Assessment Data per element type or model according to EPD or material and determining which elements have the lowest circularity. VA can also serve as a tool to support decision-making and improve the transparency of evaluation results.
The Project Data Base Module (PD-M) components are the Building Information Modelling for the Circular Economy Object Catalogue and the Element Data.
5A) The Element Data component is a spreadsheet-based documentation of all elements’ necessary LCA and Circularity assessment information. So far, data from Baubook (see Figure 2) have been implemented, but exploring further possibilities is needed. The VS-M exchanges the data with a Python script based on the chosen element type.
5B) Building Information Modelling for Circular Economy Object Catalogue: provides a pre-defined selection of different construction methods, ranging from conventional reinforced concrete construction with a thermal insulation composite system to ecologically and recycling-oriented optimised elements with detachable connections between the different layers. Achieving comparable results requires that the elements have comparable building physics properties (thermal transmittance coefficient or sound insulation parameters). The results are available, on the one hand, as element compositions and, on the other hand, in the form of a spreadsheet to assign material data to the elements. Since no properties can be assigned to compositions, it is not possible to store the data in native ArchiCAD files. The usability tests of SQL databases such as Building One are planned to increase automation,
An interface for data exchange from BA-M to VS-M is necessary to connect architectural design to graphical programming. Using the ArchiCAD-API enables access to all the relevant information about the building elements. ArchiCAD represents all building element types in one union type collection: API_Element. Each instance of API_Element has at least a header information (API_Elem_Head) and may contain extended information (memo) attached to the element. Each element stores a wide range of information, varying from geometrical data to pen information, which should be used to represent the building model and generate blueprints when the element is drawn.
The presented research using the ArchiCAD-API aimed to gather element geometry representing information. E.g., columns and beams are represented by the element’s start and end point and the cross section’s shape and may consist of many segments. Walls and slabs are represented as two-dimensional polygons (API_Polygon class) with corresponding coordinates and height parameters. Walls and slabs may contain openings, defined within the wall element itself, but the type of opening (door or window) is connected to the representing element through element-id. The mentioned elements can also contain cutting planes. The programmed Add-On collects all necessary data (provided the model was modelled according to the defined structure). It saves these as an external file accessible to the VS-M. C# scripts were implemented in VS-M, reading the data and creating the basic geometric information necessary to create the Geometric Twin, thus identifying and interpreting the collected information.
A variant study of varying construction methods is possible by linking the Geometric Twin to element properties from PD-M, so two interfaces are necessary. Firstly, the connection to the component Element Composition is made using the ArchiCAD Live Connection implemented in VS-M; this interface enables the assignment of the material composition to each element. Secondly, the connection to the component Eco-indicators is established to attribute the elements according to the chosen composition using a spreadsheet and a Python script accessing the relevant data. Consequently, the materials matching is handled within PD-M and, if conducted once, can be used for every building model. The building model is evaluated by linking geometric and alphanumeric information from BA-M and PD-M and internally calculated variables in VS-M. Evaluation examples are an LCA according to EU taxonomy and LCA and circularity assessment according to national regulations.
Further, the evaluated building variants are passed on to the CC-M using the UDP Protocol to serialise the geometry and assessment results for visualisation in the component VR Visualisation. Commands are also sent using the VA component to manipulate the VS-M and enable building design modifications. CC-M is conceptualised as a Unity-based virtual reality environment where users can view the building geometry and the evaluation metrics of the variants. The virtual agent also enables model changes, thus comparing and improving design variants and supporting decision-making.
Analysing the definition of a Digital Twin and the phases of a building’s life cycle led to the conclusion that Circular Twins need a dynamic definition along different phases (Figure 3). Since no physical object is available, the Circular Twin in the presented research is a digital twin or digital image of an already existing virtual building model − the 3D preliminary architectural design.
As a digital Twin should be able to map the entire life cycle, a rough concept was developed for the further life cycle phase according to EU reporting framework Level(s): conceptual design, detailed design and construction, as-built and utilisation services [58].
The research presented here addresses the early design stage, conducting a variant study, designing a sustainable building through a collaborative approach and providing data for further planning, construction and use. After the early design stage, the model needs to be adapted to the real/physical object during the further life cycle, on the one hand, as a data basis for resource management and, on the other hand, as an evaluation of the CT framework. During the construction phase, the model must be adapted to reflect real/used materials, thus serving as the basis for future resource management. During the use phase, the model must be kept up to date. After deconstruction, the assessment results of the design can be compared with real data, and the CT framework (especially the evaluation part) can be adapted if necessary, as shown in Figure 3.
Stages of the Circular Twin, based on different phases according to level(s)
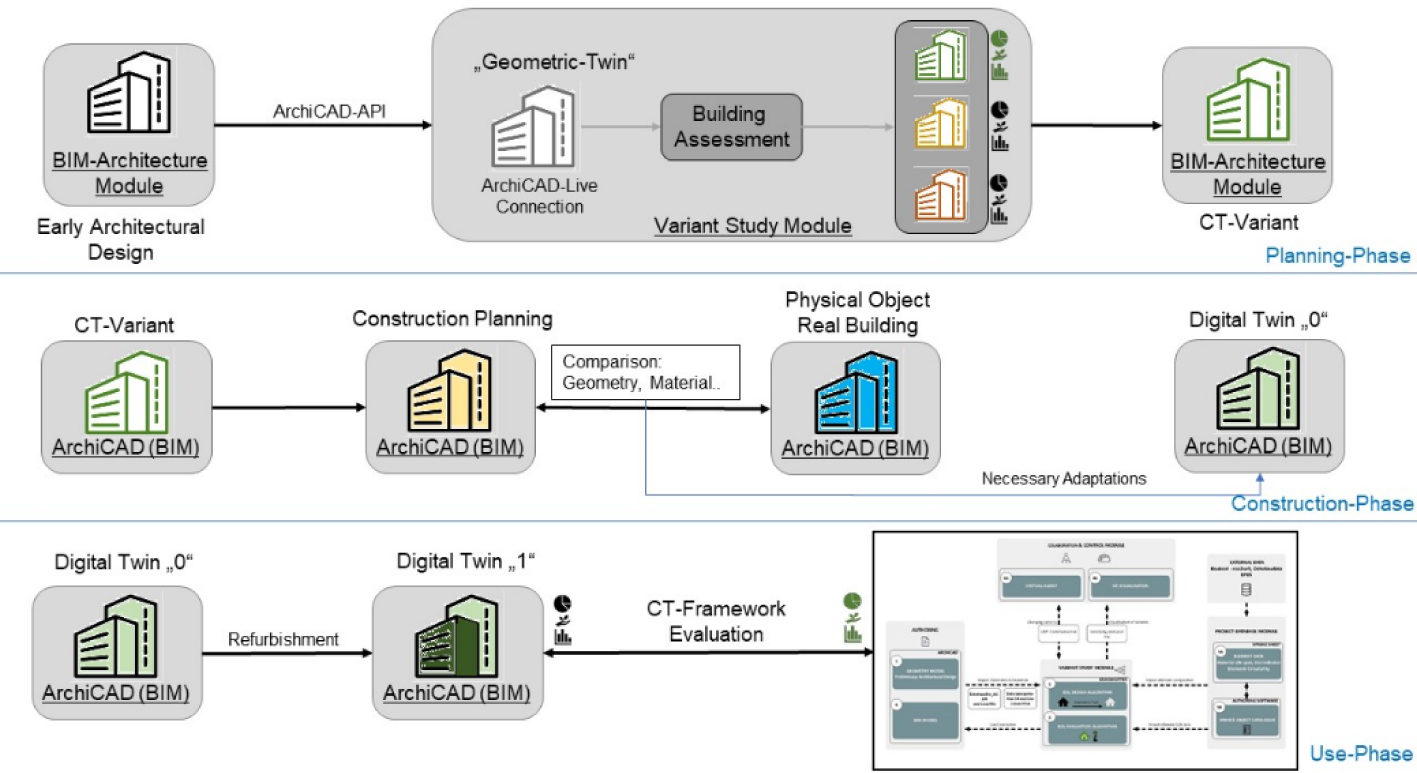
Developing the preliminary architectural design of a project and linking the geometric information to the Circular Twin framework is the first step in generating Circular Twins. By linking the geometry to the BIM4CE database, the goal is to enable the evaluation of circularity or ecological sustainability through LCA or taxonomy compliance assessment of different building variants.
The following result is achieved in a case study by testing the Circular Twin Framework using an ArchiCAD Model. Elements currently changeable are external walls, internal load-bearing walls, internal non-load-bearing walls, slabs and roofs. Two types per element are currently implemented. Each element must be placed on a specific level in BA-M, and a further level for non-changeable elements was included (e.g., in the presented use case, staircase, cellar and ground floor are not changeable). The result of collecting and interpreting data in BA-M and VS-M is shown in Figure 4.
Result of data collection in BA-M using the ArchiCAD_API and data interpretation in VS-M using C#-scripts and ArchiCAD Live Connection
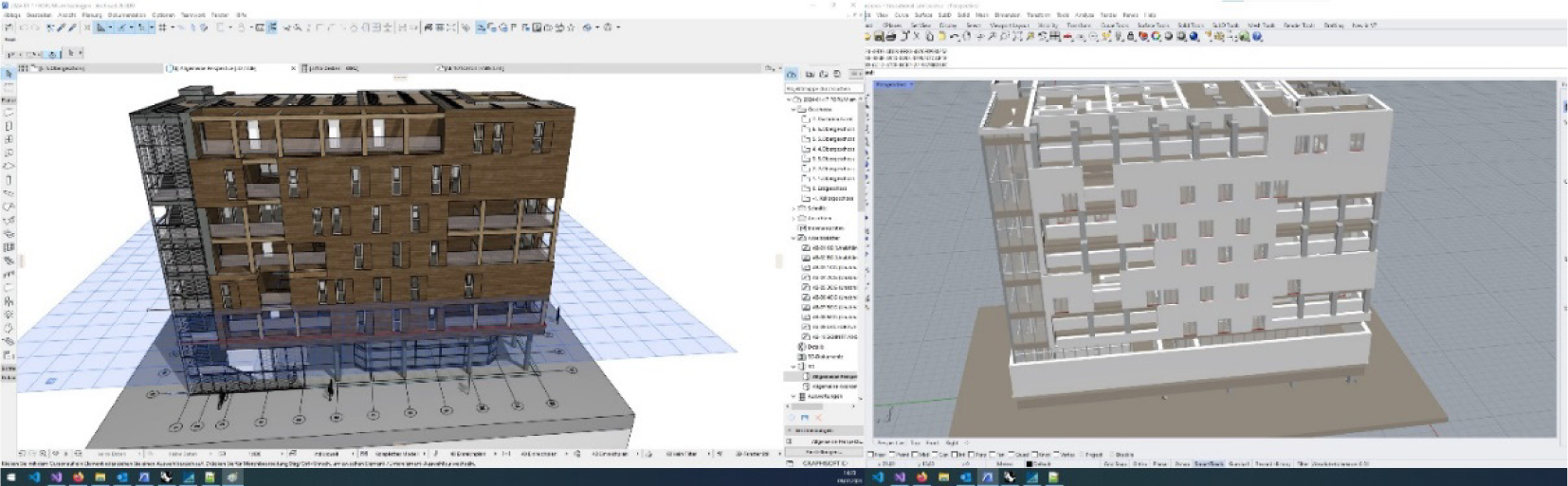
Figure 4 showcases the whole building geometry generated, and one floor, including the Element structure represented in different colours, is shown in Figure 5. The communication between VS-M and CC-M works on two tracks. On the one hand, from CC-M to VS-M via UDP connection, it transfers commands for parameter changes and from VS-M to CC-M − numerical results of the calculations. On the other hand, transferring the generated model from VS-M to CC-M is conducted by serialisation and local file exchange. The communication pipeline from CC-M to VS-M using the VA is designed in Natural Language with a virtual agent. It proceeds with automatic speech recognition (ASR), realised by NVIDIA Riva, followed by an Automatic-Speech Recognition (ASR) check to ensure the completeness and validity of the captured sentence, realised with the OpenAI GPT model.
Element-clusters generated in VS-M according to the accessible units in CC-M: Outer Walls (black), Inner Walls load bearing (yellow), Inner Walls non-load bearing (green), Slab (grey), and not changeable (red – and so far collums in orange)
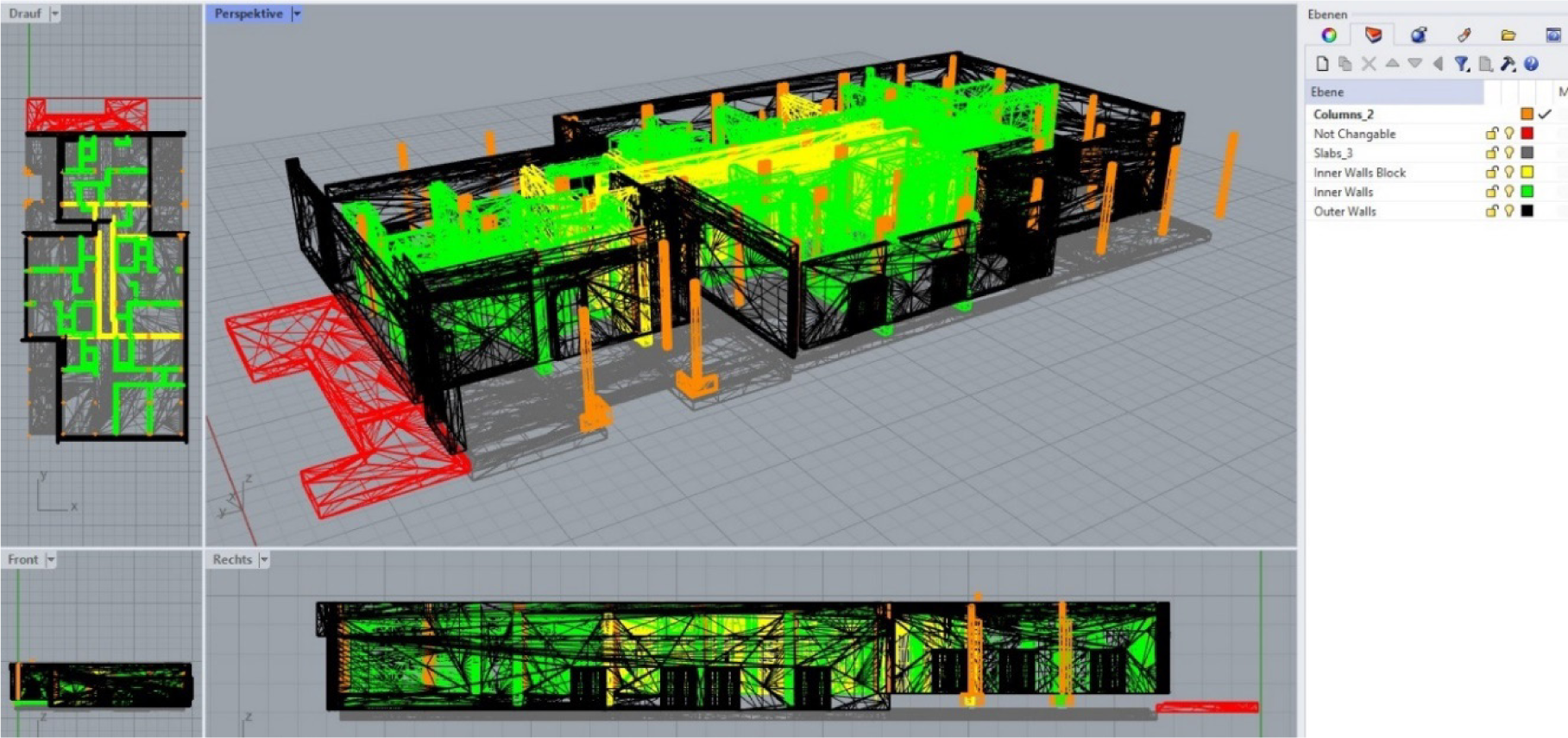
In case of incompleteness or invalidity, further processing is stopped. Otherwise, the transcribed text enters the natural language processing (NLP) phase, including understanding the natural language (i.e. identifying the desired actions to be performed) and generating natural language (i.e. generating the agent’s response to the user). The NLP phase is realised with the OpenAI GTP model. Finally, the resulting response text is converted into an audio response using Eleven Labs TTS, as shown in Figure 6.
Pipeline design for communication in Natural Language with the virtual agent and example of a query
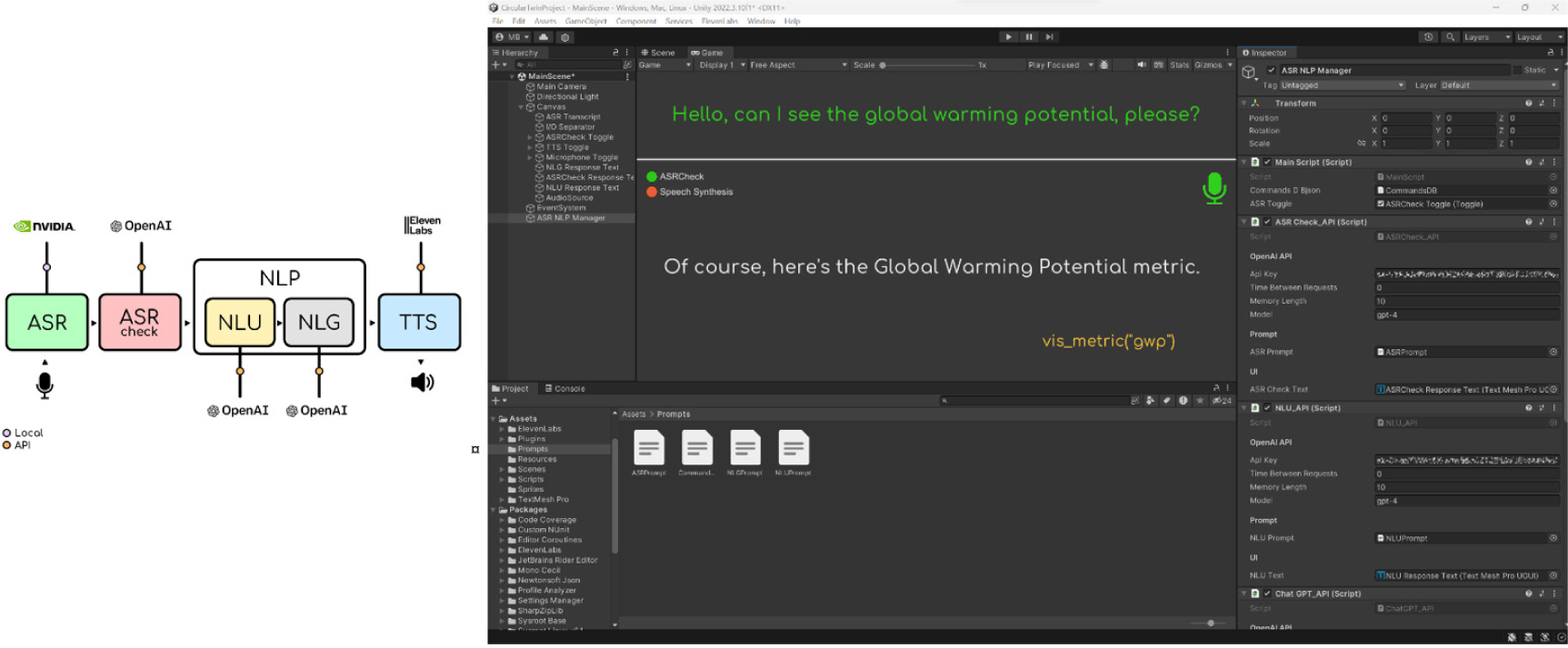
The Circular Twin Framework case study was tested in a building in Vienna (Austria) with a gross floor area of 2500 m² divided into 20 residential units and 5 office units of different sizes. Apartment sizes range from 45−93 m², all equipped with a balcony, loggia, or both and sizes of offices range from 17−193 m². It is a mixed-use building consisting of apartments, offices, and a forum on the ground floor, with a cross-site function, seven upper floors and a variation of seven different floor designs. The construction combines reinforced concrete and solid timber elements (Cross Laminated Timber – CLT), with concrete used in the access area, basement and ground floor. Planning and construction took place in the years 2018−2022.
The Variant Study was conducted by simulating the preliminary design stage by recreating a BIM Model based on the existing blueprints, collecting relevant data and sending these from BA-M to VS-M. Based on the various elements within the case study, we selected two variants for each element type (Table 1) and the element composition (Table 2) created in PD-M.
Included Elements of the Variant Study
Element | Name BA-M | Name VS-M | Name CC-M |
Outer Wall | AW03_Außenwand beheizt | OW1 | Timber Wall outside |
Outer Wall | AW05_Stgh gegen beheizt | OW2 | Concrete Wall outside |
Slab | DE05_Decke Wohnung -Wohnung | S1 | Timber Slab Parquete |
Slab | DE05b_Decke Wohnung OG | S2 | Concrete Slab |
Roof | DE06_ Extensives Gründach | R1 | Extensive Green roof |
Roof | DE07_Decke unter Freibereich | R2 | Roof terrace |
Internal Wall LB | IW01_ Wohnung - Wohnung | IW 1.1 | Double-shell Timber Wall Loadbearing |
Internal wall LB | IW03_ Gang - Wohnung | IW 1.2 | single-shell Timber Wall Loadbearing |
Internal wall NLB | IW04_LB 10cm einfach | IW 2.1 | single-shell non loadbearing |
Internal Wall NLB | LB_15cm_dreifach | IW 2.2 | Double shell non loadbearing |
Element Composition
Name VS-M | Composition (outer to inner/top to bottom) | Thickness[cm] |
---|---|---|
OW1 | Wood paneling; Fiber-cement board; 2×Wooden lathing 2.8×7.0 cm/60 cm; Vapour diffusion; Wind brake; Mineral wool between wooden battens; CLT; Vapour diffusion; Gypsum plasterboard | 3.6; 1.5; 60; 0.1; 19.5; 10; 0; 3 |
OW2 | Concrete; Mineral wool; CLT; Gypsum plasterboard; Vapour break; Gypsum plasterboard | 25; 13; 10; 1.5; 0; 15 |
S1 | Parquet flooring; Gypsum fibre screed; Impact noise insulation; Oriented Strand Board; Mineral wool between wooden battens; Oriented Strand Board; Impact noise insulation; CLT; Mineral wool soft; Gypsum plasterboard | 2; 3; 2; 2.2; 24.5; 1.5; 6; 10; 5; 3 |
S2 | Parquet flooring; Gypsum fibre screed; Impact noise insulation; Vapour diffusion; Oriented Strand Board; Mineral wool between wooden battens; Oriented Strand Board; Impact noise insulation; Concrete | 2; 3; 2; 2.2; 24.5; 1.5; 6; 30 |
R1 | Filter layer; Drainage layer; Ethylene-propylene-diene rubbers (EPDM) roof waterproofing; Slope insulation; Impact sound insulation; CLT; Mineral wool; Gypsum plasterboard; | 4; 4; 0.1; 0.1; 27; 3; 12; 5; 3 |
R2 | Wooden decking on substructure; Rubber granulate mat; EPDM roof sealing; Slope insulation; Impact sound insulation; Bituminous moisture sealing; CLT; Mineral Wool; Gypsum plasterboard | 3; 1.5; 0.2; 27; 3; 1; 12; 5; 3 |
IW 1.1 | Gypsum plasterboard; CLT; Mineral wool; CLT; Gypsum plasterboard | 3; 10; 6; 10; 3; 1.5 |
IW 1.2 | Gypsum plasterboard; Mineral wool; CLT; Mineral wool; Gypsum plasterboard | 3; 5; 10; 5; 3 |
IW 2.1 | Gypsum plasterboard; Mineral wool; Gypsum plasterboard | 1.5; 7; 1.5 |
IW 2.2 | Gypsum plasterboard; Mineral wool; Gypsum plasterboard; Mineral wool; Gypsum plasterboard | 1.5; 7; 1.5; 7; 1.5 |
Each element was combined with both variants of the other element types. Four element groups, with each two possible variants, lead to 16 building design variations in total (for each floor), as shown in Table 3. Assuming every floor is assembled with the same components, and concrete and timber are not substituted on different floors, the results, including GWP, PEI, AP, and OI3, are shown in Figure 7 and Figure 8.
Variations and the included elements considered in the Variant Study; the meaning of symbols V.X.1−16 is (Variant).(Floor Number).(Variation-Number)
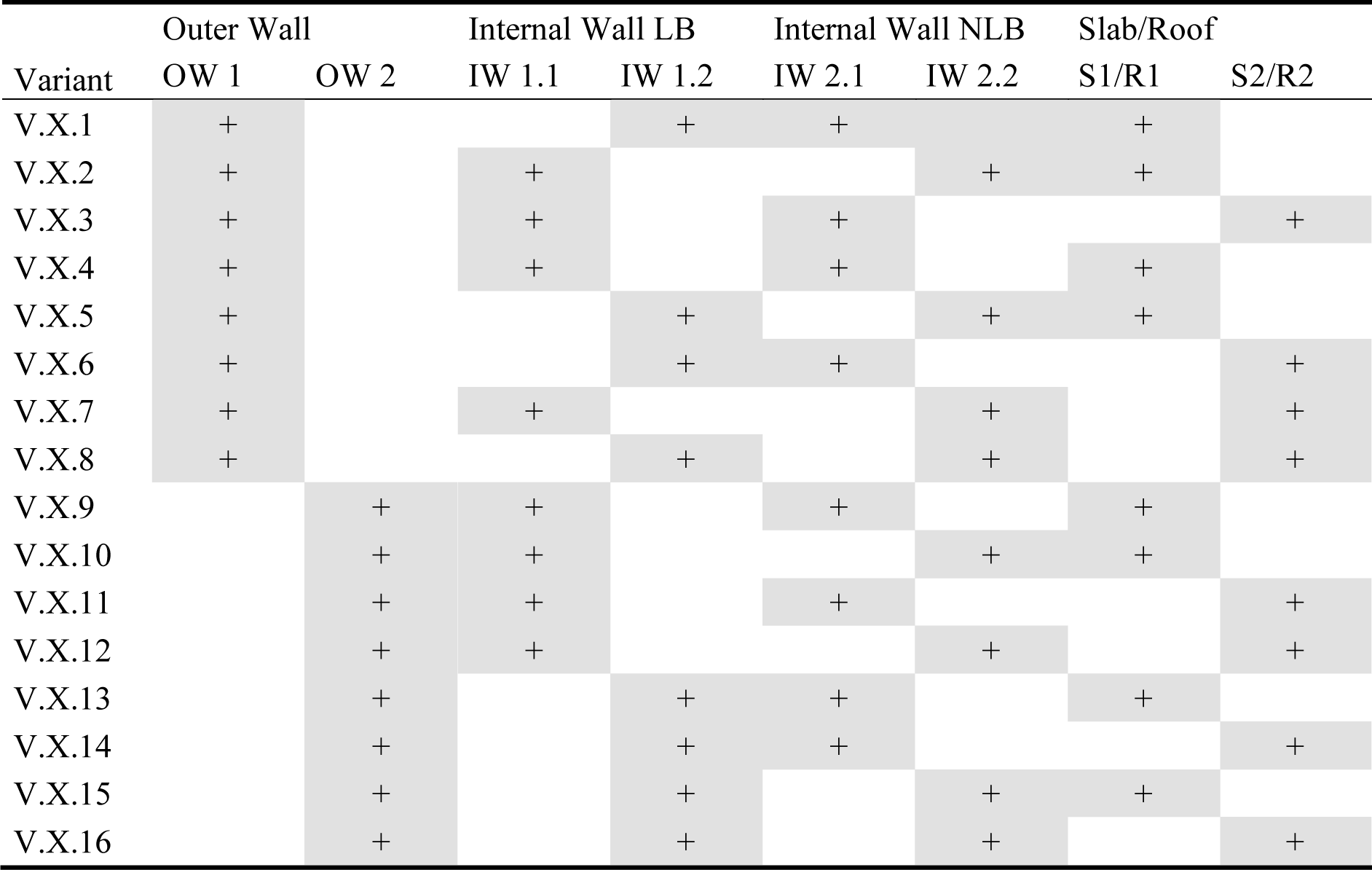
GWP and OI3-index of the 16 Variations
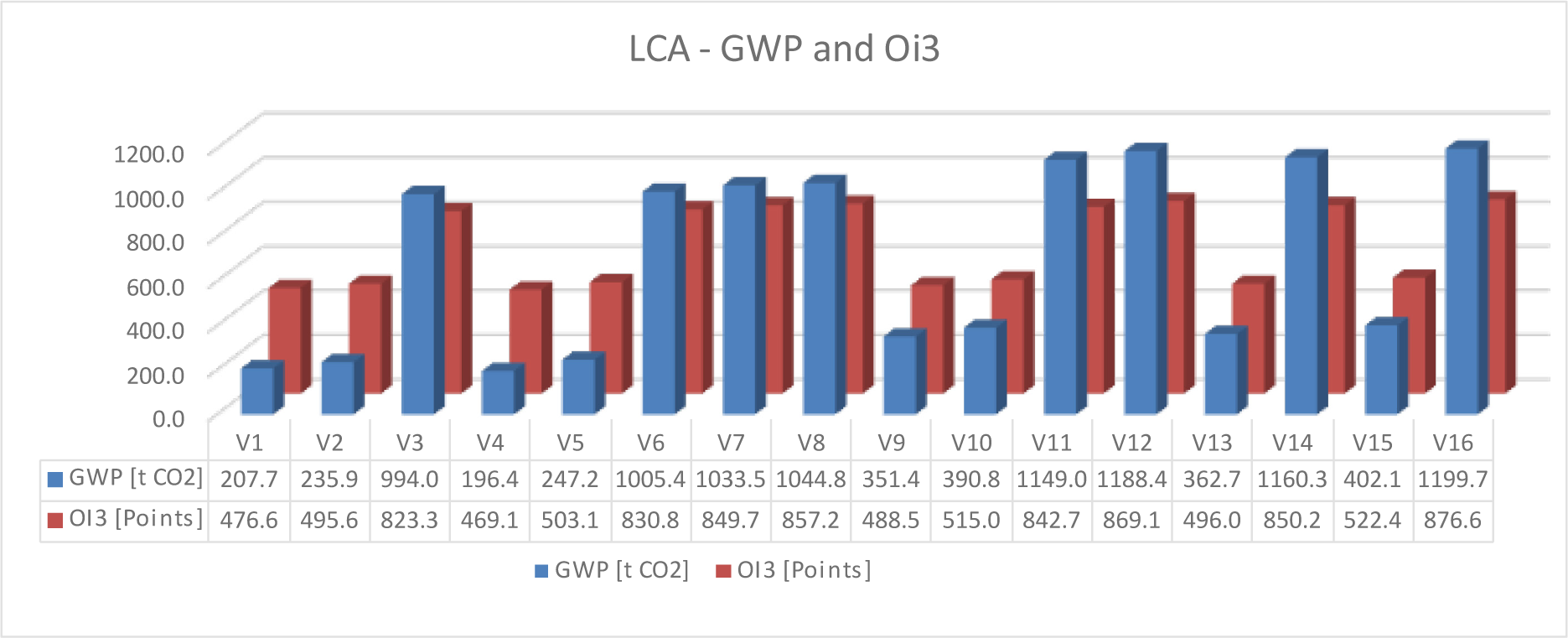
PEI and AP of the different variants
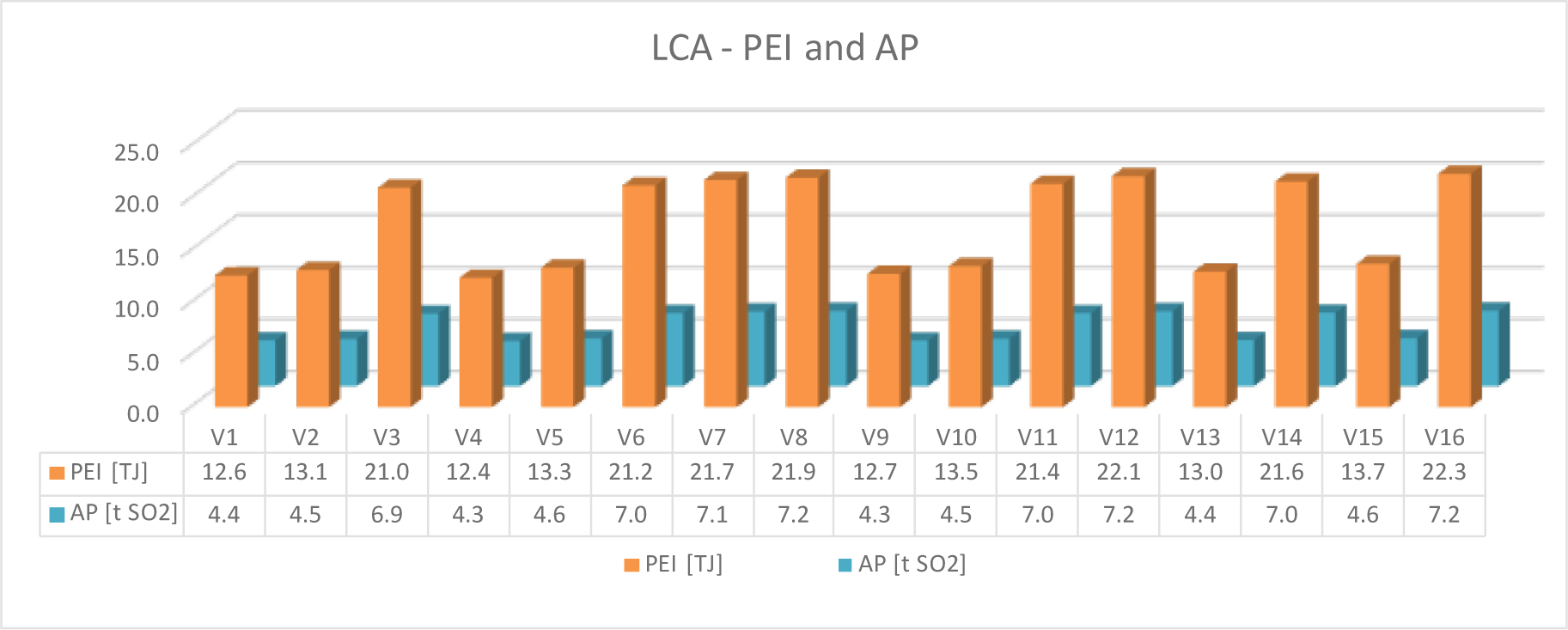
The generated geometry still contains errors, so further work needs to be done on the C# scripts to improve the interpretation of the geometry obtained from AD-M. It concerns the windows and the positioning of beams and slabs. The results of the LCA for the use case under consideration vary greatly depending on the design variant of the building. The GWP is 196−1200 t CO2, PEI of 12.4−22.3 TJ, and AP of 4.4−7.2 t SO2; if these absolute figures are used for the balancing of the entire building and the cross-floor area of the building section under consideration (staircase excluded) is 2174 m², the GWP is 90.3−551.7 kg CO2/m². The points rating also varies from 469 to 877 points according to the OI3 index frequently used in Austria. Phases A1−A3 are considered, including exchange cycles of individual element layers and a temporal balance limit of 100 years. Forming “fate families” is necessary for exchange-cycle planning because exchanging certain packages depends on the layer with the shortest service life development.
A comparison of different studies to benchmark CO2 emissions of buildings shows values in the range of 130−1350 kg CO2/m² [47]. However, the figures of the different studies vary considerably and are strongly dependent on the building typology considered, the choice of materials included, and the system limits. Since only phases A1−A3 are considered in the research presented here, variants with the lowest GWP have a very high proportion of wood (which has a negative carbon footprint in these phases); this explains why all four studies fall below the minimum values. Including all phases according to the EPD, circularity assessment and evaluation of material efficiency are currently under development.
The presented research under the barriers listed in the studies indicates that the Circular Twin digital ecosystem can be seen as an incubator for the implementation of CE in AEC. Listed barriers and the associated levels are, for example, lack of investment in promoting circularity in construction (Political), cost and schedule constraint-tight budget (Economic), designer’s leadership role, and lack of stakeholders’ awareness of the benefits of circularity in construction (Social). Worth mentioning are the information gap, supply and demand matching, lack of expertise and knowledge in circularity in construction, complexity of the design process (Technological), lack of coordination between stakeholders (Legal), accurate quantification of the environmental benefits, and tradeoffs between different sustainability strategies (Environmental) [19]. The influence on the different barrier levels targeted with the presented research is as follows:
Political/Economic: A cost estimation could easily be implemented in the presented framework, enabling an assessment of improvement of environmental performance per investment. Such a tool could also serve as a promoting tool, showcasing the best improvement measures.
Social: The collaborative VR platform visualising assessment results and different design variants and discussing them interdisciplinary is very promising in raising awareness and explaining designs to non-expert persons, thus increasing the designer’s leadership role.
Technological: Using a BIM approach allows for the provision and use of relevant information in a continuous data chain. One of the major problems in implementing high-level CE measures is the lack of information regarding the material composition of buildings; the presented research supports the reduction of this barrier.
Environmental: The presented framework makes it possible to assess the impact of construction activities on the environment as early as the planning stage. It also provides data on the further life cycle, promoting recycling and, thus, reducing resource requirements.
It should be mentioned that when bridging technological barriers, care must be taken to ensure that the people who use them also have the necessary skills to apply them. Looking at the impact of the research presented in this paper on the three pillars of sustainability, the positive influence on environmental sustainability through improved emissions and more resource-efficient design is obvious.
However, this also results in other effects regarding the lower land requirement due to the reduction in the volume requirement of the landfill, which has indirect positive effects on biodiversity due to less intervention in existing ecosystems. However, there are also economic advantages that derive from the establishment of CE in the AEC industry. For example, gaining economic potentials, such as creating new economic sectors and occupational fields, which entails increased employment and a regional value chain with direct and indirect effects, is very welcome from a financial perspective [14], [59]. The development and establishment of new business models can be named as examples. Waste management follows two approaches: the elimination of contaminated sites and the creation of functioning waste systems.
Part of these business models is based on the fact that promoted building design will require higher manual effort for dismantling, and the recovered materials must be processed and returned to cycles, which leads to an increase in jobs, as well as resource security, and prevents dependence on imports and the associated risk of price fluctuations.
The framework presented in this paper describes a collaborative design tool concept for the early planning stages of a building´s design to support decision-making and stakeholder collaboration. The aim is to create Circular Twins to assess design variants for their sustainability performance regarding recyclability, environmental impact and compliance according to EU taxonomy. Establishing a VR platform and supporting a VA represents a novel approach in the construction industry.
The research so far shows that the software landscape is extensive and has the desired functionalities of an island. However, there is a lack of interoperability, especially in consistent data management. In the wake of climate change and the ever-increasing demand for a circular economy, and considering the influence of the AEC industry, it is necessary to provide planners and stakeholders with appropriate planning tools. By linking different tools, it is possible to quickly evaluate design variants, graphically illustrate them, and increase design quality.
Essential for further research is the design of the interfaces, like the investigation of open format interfaces, such as IFC as data transfer of the architectural design, as well as the serialising process as communication between Grasshopper and VR, to increase the use of open source. Furthermore, the scope of the assessments needs to be expanded so all phases are accounted for and in more detail to discuss individual phases in VR. Expanding circularity assessment to investigate the interactions between circular material use and emissions reduction is crucial for promoting CE. The goal must be to design buildings that perform well in both fields.
Another point is that the VA must be able to understand and explain the evaluation metrics and, if necessary, point out the potential for improvement. For this purpose, as well as for the overall operation of the digital ecosystem in a VR environment, user studies are carried out asking how intuitive the operation is and whether the presentation of results is understandable.
This research was conducted within the research project “Circular Twin - Ein digitales Ökosystem zur Generierung und Bewertung kreislauffähiger Digitaler Zwillinge” (in English: Circular Twin − A digital ecosystem for generating and evaluating circular digital twins), which received funding from the Austrian Ministry for Climate Action, Environment, Energy, Mobility, Innovation and Technology through the Austrian funding agency (FFG). Grant number: 899167. through which the conduction of this research was enabled.
AAD | Algorithm Aided Design |
AEC | Architecture, Engineering and Construction |
AP | Acidification Potential |
BA-M | BIM Authoring Module |
BIM | Building Information Modelling |
BIM4CE | Building Information Modelling for Circular Economy |
CC-M | Collaboration and Control Module |
CE | Circular Economy |
DGNB | Deutsche Gesellschaft für nachhaltiges Bauen |
EoL | End of Life |
EPD | Environmental Product Declaration |
EPDM | Ethylene-propylene-diene rubbers |
EU | European Union |
GWP | Global Warming Potential |
LCA | Life Cycle Assessment |
LEED | Leadership in Energy and Environmental Design |
PD-M | Project Database Module |
PEI | Primary Energy Input |
PESTLE | Political, Economic, Social, Technological, Legal, and Environmental |
UDP | User Datagram Protocol |
VA | Virtual Agent |
VR | Virtual Reality |
VS-M | Variant Study Module |
- What a Waste 2.0 - A global Snapshot of solid Waste Management to 2050Washington, 2018,
- Life cycle assessment of building materials: Comparative analysis of energy and environmental impacts and evaluation of the eco-efficiency improvement potential, 2011,
- Recycling value of building materials in building assessment systems, Energy and Building, 2011,
Service contract on management of construction and demolition waste-SR1 Final Report Task 2 , 2011
, - Digital Platform for Circular Economy in AEC Industry, 2020,
Buildings as Material Banks and the need for innovative Business Models, (Technical Report) , 2017
, - , Building a Circular Future, 3rd-Edition, 2018
Materials Passports - Best Practice ,Report , 2019
, United Nations Environmental Programm (UNEP) - 2020 Global Status Report for Buildings and Construction: Towards a Zero-emission, Efficient and Resilient Buildings and Construction Sector. Report, United Nations Environment Programme (UNEP) (2020) ,
, - The Circular Economy – A new sustainability paradigm?, 2017,
- Report of the World Commission on Environment and Development: Our Common Future Towards Sustainable Development 2. Part II. Common Challenges Population and Human Resources 4, 1985, https://sustainabledevelopment.un.org/content/documents/5987our-common-future.pdf
- Principal sustainability components: Empirical analysis of synergies between the three pillars of sustainability, 2012,
- Sustainable Development Goals - SDG, 2021, https://sdgs.un.org/goals
- , Volkswirtschaftliche Effekte durch Recycling ausgewählter Altstoffe und Abfälle, Recycling und Rohstoffe, 2018
- 4D-BIM to enhance construction waste reuse and recycle planning: Case studies on concrete and drywall waste streams, 2020,
Bundesgesetz über eine nachhaltige Abfallwirtschaft (Abfallwirtschaftsgesetz 2002 – AWG 2002). BGBl. I , 2002
, - , How Network Governance Powers the Circular Economy Ten Guiding Principles for Building a Circular Economy, Based on Dutch Experiences, 2020
Circular economy principles in construction ,Report , 2020
, - Barriers To Circularity In Construction: An Analysis Of Experts’ Perspectives, 2023,
- Evaluating Barriers, Enablers and Opportunities for Closing the Loop through ‘Waste Upcycling’: A Systematic Literature Review, 2022,
- Describing the changes in architectural information technology to understand design complexity and free-form architectural expression, 2006, http://itcon.org/2006/29/
From BIM towards Digital Twin: Strategy and Future Development for Smart Asset Management ,Proceedings of SOHOMA 2019 , 2020
, - Computational Algorithms for Digital Twin Support in Construction, 2020,
- Parametric and Generative methods with BuildinG information modellinG Connecting BIM with explorative design modelling, 2012,
- The hype factor of digital technologies in AEC, 2021,
- Digital Twin Research in the AECO-FM Industry, 2021,
- Digital twin driven energy-aware green design, 2020,
- Parametric design and BIM, systemic tools for circular architecture, 2019,
- Visualisation in architecture, engineering and construction (AEC), 2005,
- Supporting constructability analysis meetings with Immersive Virtual Reality-based collaborative BIM 4D simulation, 2018,
- Chances and Limitations of a Virtual Reality-supported Tool for Decision Making in Industrial Engineering, 2018,
- Parametric Modelling Within Immersive Environments Building a Bridge Between Existing Tools and Virtual Reality Headsets, 2018,
- BIMFlexi-VR: A Virtual Reality Framework for Early-Stage Collaboration in Flexible Industrial Building Design, 2022,
Virtual humans and persuasion: The effects of agency and behavioral realism ,Media Psychology , 2007
, - Virtual reality exposure therapy for adolescents with fear of public speaking: A non-randomised feasibility and pilot study, 2019,
Detection and computational analysis of psychological signals using a virtual human interviewing agent ,Journal of Pain Management , Vol. 9 , 2016
, - It’s only a computer: Virtual humans increase willingness to disclose, 2014,
- Harnessing the power of conversations with virtual humans to change health behaviors, 2016,
- Building Intelligent Virtual Agents as Conversational Partners in Digital Construction Sites, 2018,
- An integrated BIM-LEED application to automate sustainable design assessment framework at the conceptual stage of building projects, 2020,
- ÖNORM EN ISO 14040 - Environmental management-Life cycle assessment-Principles and framework, 2021, www.austrian-standards.at/webshop
- ÖNORM EN ISO 14044 - Environmental management-Life cycle assessment-Requirements and guidelines, 2021, www.austrian-standards.at/webshop
- Verifying the Environmental and Energy Feasibility of Potential Improvement Actions in the Steel Production Chain in Brazil, 2022,
ÖNORM EN 15978 - Sustainability of construction works - Assessment of environmental performance of buildings - Calculation method , 2012
, - www.ibo.at. , 2018,
- Life cycle assessment in buildings: The ENSLIC simplified method and guidelines, 2011,
- Environmental benchmarking of building typologies through BIM-based combinatorial case studies, 2021,
- Material Circularity Indicator (MCI), https://www.ellenmacarthurfoundation.org/material-circularity-indicator
- , Urban Mining Index Entwicklung einer Systematik zur quantitativen Bewertung der Kreislaufkonsistenz von Baukonstruktionen in der Neubauplanung, 2020
- www.ibo.at , 2018,
- Material Passports and Circular Economy, in: An Introduction to Circular Economy, 2020,
- Improving the recycling potential of buildings through Material Passports (MP): An Austrian case study, 2019,
- Entwicklung eines Konzepts zur Förderung der Kreislaufwirtschaft im Bauwesen: Materieller Gebäudepass und Design for Recycling für das Bauwesen Endbericht, (technical) Report 2011, www.iwa.tuwien.ac.at
- Improvement of Environmental Sustainability and Circular Economy through Construction Waste Management for Material Reuse, 2022,
- Assessment of sustainable use of material resources in the Architecture, Engineering and Construction industry - a conceptual Framework proposal for Austria, 2022,
Sustainable Construction: Green Building Design and Delivery , 2022
, - From Sketching to BIM Workflow for the Generation of IFC-based BIM Models from 4D Semantic Sketches, 2023,
- Level(s) - European framework for sustainable buildings, https://environment.ec.europa.eu/topics/circular-economy/levels_en
The European Green Deal. Communication From The Commission To The European Parliament, The European Council, The Council, The European Economic And Social Committee And The Committee Of The Regions, COM(2019) 640 final 2019 ,
,